Confocal Microscopy Techniques
Confocal Raman and Luminescence Microscopy at Room Temperature

Optical set-up of our confocal Raman microscope.

Part of the facet eye of a bumble-bee as imaged with the confocal microscope (image size, 55 × 55 µm).
In a confocal microscope, a laser beam is focused to a diffraction-limited spot on the sample with a microscope objective of high Numerical Aperture. Light scattered from this spot in backward direction is collected and collimated by the same objective and focused through a tiny pinhole onto a photodetector. Only light from the focus can penetrate through the pinhole; light from other depths in the sample is efficiently blocked.

Surface structures of an older EPROM chip as seen in elastically scattered laser light (left) and in the light of the Si Raman line at 520 cm-1 (image size, 100 × 100 µm).
As a consequence, out-of-focus regions do not blur the signal, but are dark. This provides the possibility to investigate different depth layers separately and to obtain three-dimensional microscope images. In contrast to a regular microscope, the sample must be raster-scanned with respect to the laser focus (or vice versa), however, since only one point is illuminated at a time. Raster-scanning can be accomplished with a three-piezo stage.

Three-dimensional arrangement of the grains of artificial Carlsbad salt as recorded with confocal Raman microscopy. Spatial distributions of SO42- (ν = 985 cm-1; left) and HCO3- ions (ν = 1033 cm-1; right).
With a confocal optical set-up it is not only possible to detect elastically scattered light, but also other types of secondary radiation emerging from the laser focus. Examples are fluorescence, different types of photoluminescence, Raman scattering, or - in the case of nonlinear-optical materials - higher harmonics.
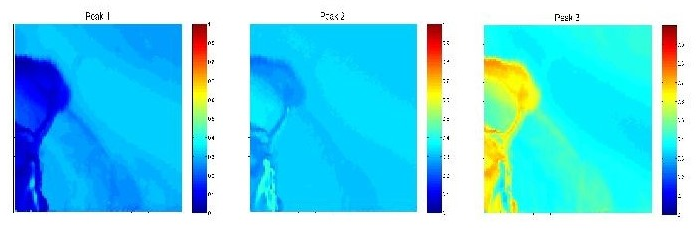
Spatial distribution of three different crystal modifications at the surface of a GaSe single crystal. The modifications were identified according to the emission wavelengths of their direct-gap Wannier excitons (image size, 100 × 100 µm).
In this way the instrument operates as a confocal luminescence or Raman microscope. The different frequency components of the collected light are dispersed in a monochromator, whose entrance slit is replaced with the pinhole. A CCD line detector is placed in its exit focal plane so the whole spectrum is recorded in parallel.

Cross sections of spider silk fibers of two different spider species. The fiber shown in the left picture is clearly hollow, whereas the one in the right panel is compact. The intensities of characteristic Raman lines are plotted in false-color representation.
Measuring the integrated intensity of characteristic Raman or luminescence lines as a function of the focal position yields a three-dimensional image of the chemical composition of the sample by purely optical means in a non-destructive fashion.
Confocal Fluorescence Lifetime Imaging Microscopy (FLIM)

Optical set-up of our FLIM apparatus.

Fluorescence amplitude (left) and lifetime (right) of fluorescent polymer micro-spheres.
Regular fluorescence microscopy can be extended to measure the fluorescence lifetime(s) at each spatial position. This is known as fluorescence lifetime imaging microscopy (FLIM). Our set-up works in the frequency domain. The cw laser which is used for excitation is amplitude-modulated in the range of several ten MHz with an acousto-optic modulator (AOM), and the modulated fluorescence is detected phase-sensitively. The non-zero fluorescence lifetime leads to a phase shift and a decreased modulation amplitude of the fluorescence signal with respect to the excitation. The lifetime can be calculated from both effects.